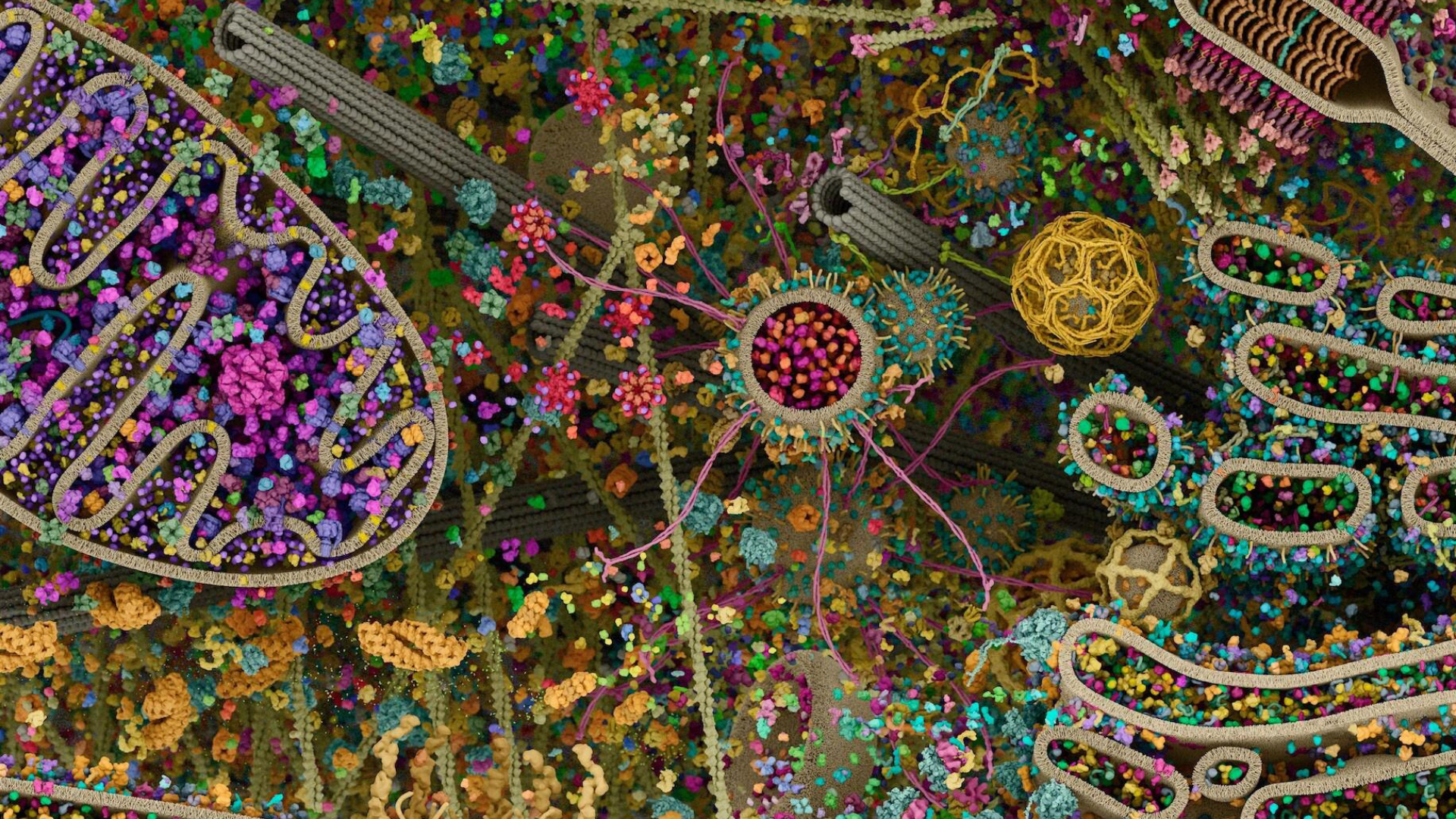
Our laboratory studies the underlying mechanisms of degenerative diseases such as brain degeneration. To better understand the molecular mechanisms of life processes and how they are corrupted by disease, our work focuses on the conserved pathways that control the function of subcellular compartments in cells. We utilize a multi-disciplinary approach that bridges detailed biochemical and cellular studies with molecular imaging and perform robust quantitative analysis of critical cellular mechanisms. The malfunction of essential cellular machines underlies a plethora of errors in key physiological processes and strongly contributes to the development of a growing list of metabolic diseases, neurodegenerative disorders, and age-related illnesses. The long-term goal of our laboratory is to develop innovative approaches to understand how cellular machines function at biochemical depth and decipher the links between cellular dysfunction and its relationship to metabolic and neurological disorders.
Why do mitochondria matter?
Disease development is associated with a general functional decline in physiological activity, including mitochondrial processes. There is a growing understanding of how metabolic alterations, oxidative stress, DNA damage, inflammation, calcium dyshomeostasis, and several other factors may underlie the decline in cellular dynamics and function. For instance, the progressive deterioration of brain cell structure and function is often accompanied by mitochondrial dysfunction, impacting key cellular processes ranging from metabolism and signaling to differentiation and programmed cell death. Yet, we do not understand the mechanistic links between mitochondrial dysfunction, neurodegeneration, and nervous system pathologies. Investigating the timing, compartmentalization, communication, and localization of mitochondrial processes is critical for advancing our understanding of neuronal physiology, degeneration and regeneration, and may also offer potential intervention strategies.
How do cells communicate to improve health and viability?
The transfer of information between cells has been shown to facilitate metabolic coupling, resolve inflammatory pain, contribute to neuro-recovery mechanisms after stroke, and enhance tumorigenesis. How do cells decide which molecules should be released into the extracellular space? What are their functional roles upon internalization by recipient cells? Although no universal mechanism may exist, our goal is to characterize key intra- and extra-cellular processes to provide a better understanding of precise signatures essential for intercellular communication.
To achieve this, we aim to develop a biochemical framework that enables molecular characterization of cellular machines and complement this with high-resolution imaging techniques to investigate the key interactions facilitating the large remodeling of the cellular landscape. These efforts will help identify the molecular bases of human pathologies associated with cell-cell communication and increase the probability of success in developing new therapeutic approaches.
How do lipids regulate cellular function?
Our lab tackles a central challenge in cell biology: understanding how lipids cooperate with proteins to self-assemble into membranes of mutable shapes that define the organizational principles of cellular systems. Cells orchestrate spatial and temporal organization of critical biochemical processes to enable multiple, often competing, reactions to occur simultaneously. This cellular complexity is driven by lipid and protein catalysts, which play key roles in the modulation of cellular architecture, metabolism, and signaling. Thus, our research is focused on understanding the native principles of membrane dynamics in eukaryotic and bacterial systems. We combine molecular imaging and mechanistic investigations and develop novel approaches, including new visualization tools for monitoring lipid-lipid and lipid-protein interactions and unique in vitro reconstitutions to engineer complex biochemical processes in human and bacterial cells. This combination of experimental methods allows us to overcome the genetic, biochemical, and computational challenges to study dynamic processes in molecular detail and provide a critical foundation for understanding the mechanisms connecting lipids and cellular dynamics and function.